Neue Glasfaser-Teststrecke für Quantennetzwerke
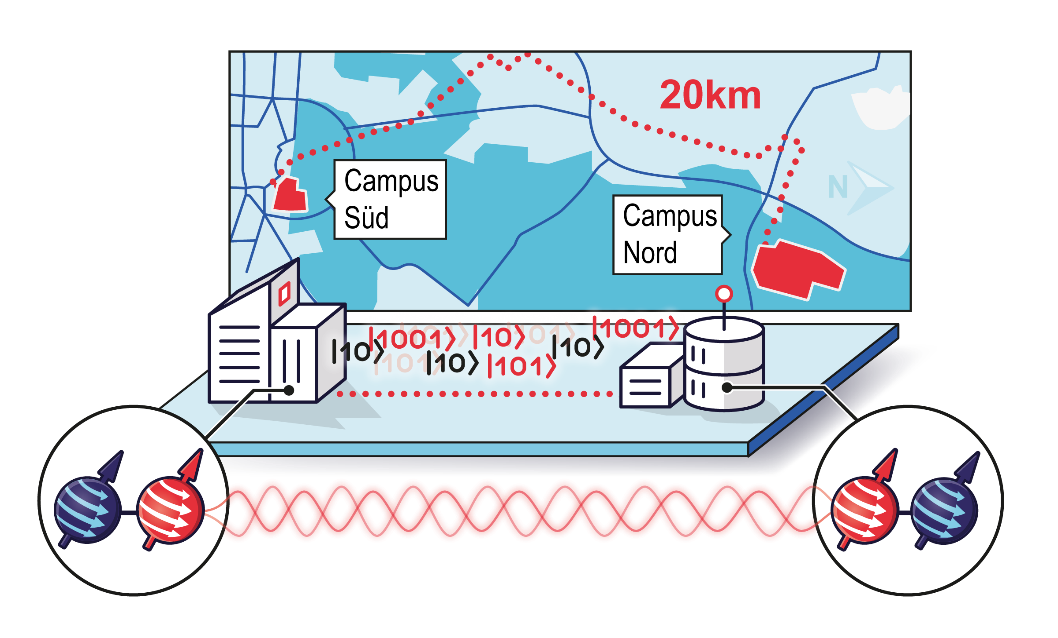
Abhörsichere Kommunikation ist für eine vernetzte Gesellschaft von zentraler Bedeutung. Entsprechende Grundlagen liefert die Quantenphysik: Sie ermöglicht praxistaugliche Technologien wie die Quantenschlüsselverteilung. Mit der neuen Glasfaser-Teststrecke am Karlsruher Institut für Technologie (KIT), die am Mittwoch, den 22. Januar 2025, eröffnet wurde, wollen die Forschenden solche Schlüssel übertragen, testen und weiterentwickeln. Darüber hinaus wollen sie ein Quantennetzwerk aufbauen mit dem Quantenverschränkung übertragen werden kann. Damit soll die Reichweite von Quantenkommunikation erweitert werden und die Verknüpfung von Quantencomputern ermöglicht werden.
Mit der neuen Glasfaser-Teststrecke nutzen die Forschenden modernste Technologien, wie hochkohärente Laser, um die Quantenschlüssel zu erzeugen und zu übertragen. Diese sind entscheidend für eine abhörsichere Kommunikation, da sie auf physikalischen Gesetzen basieren und nicht wie bisherige Schlüssel auf mathematischen Annahmen, die mit künftigen Quantencomputern gebrochen werden können.
Mit einer Länge von 20 Kilometern verbindet eine von der Firma TelemaxX angemietete Glasfaser als quantenoptische Übertragungsstrecke speziell ausgestattete Labore mit aufwändigen Lasern und Kryostaten am Campus Süd und Campus Nord des KIT. Der lichtleitende Kern der Glasfaserleitung hat einen Durchmesser von nur etwa 9 Mikrometern. Ein menschliches Haar ist im Vergleich dazu etwa 60 Mikrometer dick.
„Mit der errichteten Teststrecke stellt das KIT den Forschenden eine wichtige Infrastruktur zur Verfügung, um an den Möglichkeiten der Quantenphysik zu forschen“, sagt Professor Oliver Kraft, Vizepräsident Forschung des KIT. „So leistet das KIT einen entscheidenden Beitrag, um Quantentechnologien voranzutreiben und hin zu Anwendungen zu bringen.“
„Wir haben nun mit der Glasfaser- Teststrecke eine wichtige Plattform, um die Quantenschlüsselverteilung weiterzuentwickeln, grundlegende Charakterisierungen durchzuführen und in die klassische Kommunikation zu integrieren“, sagt Projektleiter Professor David Hunger vom Physikalischen Institut (PHI) am KIT. „Darauf aufbauend wollen wir außerdem neuartige Übertragungsprotokolle für die Schlüssel entwickeln. Zum einen wollen wir Methoden entwickeln um Quantenkryptographie effizienter und praktikabler zu machen. Hierzu arbeiten wir z.B. mit der Firma KEEQuant zusammen, einem Startup-Unternehmen auf dem Gebiet der Quantenkryptographie. So konnten wir bereits erste Quantenschlüssel zwischen Campus Nord und Campus Süd austauschen. Zum anderen wollen wir mit Hilfe spezieller Materialien wie z.B. molekularer Quantenemitter und Farbzentren in Diamant hochreines Quantenlicht – einzelne Lichtteilchen, oder sogenannte Photonen – erzeugen und damit Übertragungsraten erhöhen.“
Aufbau eines Quantennetzwerks
Zusätzlich wollen die Wissenschaftlerinnen und Wissenschaftler in mehreren Schritten ein Quantennetzwerk aufbauen mit dem sie Elemente des künftigen Quanteninternets erforschen. Zwei zentrale Schritte dahin sind die Speicherung von Quanteninformation und die quantenmechanische Verschränkung von Quantenspeichern. Damit können z.B. sogenannte Quantenrepeater realisiert werden, mit deren Hilfe Quanteninformation auch über große Distanzen übertragen werden kann. Verschränkung ist auch ein Grundelement von Quantencomputern, und optische Übertragung von Verschränkung ermöglicht die Verknüpfung von Quantencomputern in einem Quanteninternet.
Teststrecke als Teil der Exzellenzcluster-Initiative Chem4Quant
Das Projekt ist eine zentrale Infrastruktur der Exzellenzcluster-Initiative Chem4Quant in der Forschende des KIT, der Universität Ulm und der Universität Stuttgart gezielt Materialstrukturen für künftige Quantentechnologien aufbauen wollen und sich auf ein neues Teilgebiet der Quantentechnologien, die molekularen Quantensysteme, fokussieren. Zudem ist es eine wichtige Komponente für die Forschung zu Quantenrepeatern im Rahmen des BMBF Verbundprojekts Quantenrepeater.Net (QR.N), sowie ein Beitrag für den Baden-Württemberg-weiten Innovationscampus QuantumBW.
Ansprechpartner:
Prof. Dr. David Hunger, Physikalisches Institut
Prof. Dr. Sebastian Randel, Institut für Photonik und Quantenelektronik
Research topics
One of the central goals is to enhance light-matter interactions to realize efficient light-matter interfaces at the single quantum level, and to enable novel schemes for spectroscopy and sensing. We employ and further develop fiber-based Fabry-Perot microcavities, which combine microscopic mode volumes with exceptionally high quality factors, and at the same time offer open access for a variety of samples.
Spin-photon interface for single rare earth ions
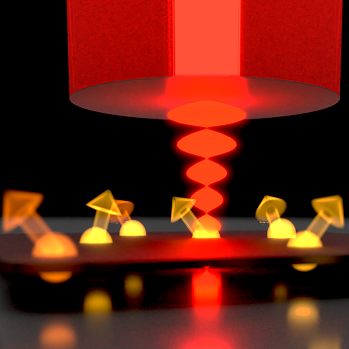

Rare earth ions provide exceptional optical and hyperfine coherence, which makes them promising candidates for quantum information applications, ranging from quantum memories and quantum computing nodes to quantum-nonlinear optics. However, the dipole-forbidden nature of the narrow transitions results in ultra-low emission rates, limiting most experiments to macroscopic ensembles. We want to gain efficient access to individual ions and small ensembles by coupling them to a high finesse optical microcavity. Purcell enhancement can boost the emission rate by several orders of magnitude, thereby making the weak transitions bright and enabling efficient state readout. We use microcavities based on laser-machined optical fibers as mirror substrates, which combine high finesse with small mode cross sections and full tunability.
The fiber-opposing planar mirror incorporates the rare-earth ion doped nanocrystals in the cavity (Fig.1). Addressing of individual ions is achieved in the spectral domain via a stabilized, narrowband laser. This scheme makes use of the large ratio between the broad inhomogeneous crystal linewidth versus a narrow homogeneous single ion linewidth (Fig.2) opening up the possibility to have efficient access to possibly thousands of qubits contained in one cavity mode.
As a first step, we have performed cavity-enhanced fluorescence spectroscopy of individual nanocrystals, allowing the detection of signals from small ensembles of about 10 Europium ions [Casabone et al.]. We were able to demonstrate multi-modal Purcell enhancement and to measure the homogeneous linewidth of small ensembles within a single nanoparticle [Eichhorn et al. 2024]. Within a collaboration with Hugues de Riedmatten, this approach could be used to demonstrate strong and dynamically switchable Purcell enhancement of Erbium ions in a nanocrystal [Casabone 2020]. Harnessing emission enhancement by up to hundred-fold, also single Erbium ions with narrow linewidths could be observed [Deshmukh et al. 2023].
Molecular lanthanide complexes
Recently, we started to study molecular complexes based on Europium which show remarkable optical coherence and long-lived nuclear spin states [Serrano 2022, Kuppusamy 2023 Schlittenhardt 2024, Kuppusamy 2024]. Synthetic chemistry opens up the possibility to tailor the optical and spin properties, such that optimized photon emission and spin coherence can be specifically targeted. Furthermore, molecules can be arranged in regular arrays such as molecular crystals or supra-molecular assemblies, e.g. via self-assembly. This opens up the perspective for the controlled realization of scalable qubit registers.
Spin-photon interface for color centers in diamond
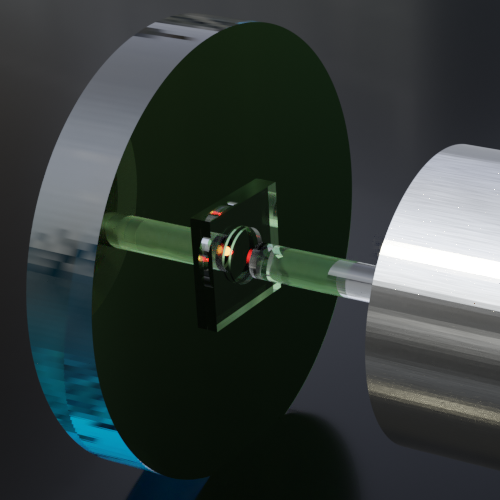
Building a long distance quantum network is one of the big challenges in the field of quantum communication, which requires the development of a quantum repeater. A crucial component of this is an efficient, coherent spin photon interface, and coupling single color centers in diamond to a microcavity is a promising approach therefore. In our experiments, we integrate a diamond membrane to an open access fiber-based Fabry-Perot microcavity [Heupel et al., Micromachines 2020] to attain emission enhancement into a single well-collectable mode as well as spectral filtering. Simulations predict the feasibility of a strong enhancement of the Zero-Phonon-Line (ZPL) emission efficiency, reaching values of up to 80%. We have performed spatially resolved characterizations of different coupled cavity-membrane devices and observed significant effects of the diamond membrane on the cavity mode properties [Körber et al]. We have also integrated such devices in a cryogenic environment and achieved highest mechanical stability for operation under resonance conditions [Pallmann et al. 2023].
Recently, in collaboration with Wolfgang Wernsdorfer and Christoph Becher, we have started to study the SnV center in diamond as a promising alternative. We have observed Fourier-limited and long-term stable optical transitions, long spin lifetime at sub-K temperature, and achieved coherent spin control by straining the diamond as well as by using a superconducting waveguide to avoid heating due to dissipation. This allowed us to observe electron spin coherence of up to 10ms under dynamical decoupling [Karapatzakis et al., 2024].
Cavity-enhanced sensing platform in a microfluidic cell
The detection and characterization of individual non-fluorescent nanosystems with high temporal resolution could provide new insights into their intrinsic properties and dynamics. To achieve the high sensitivity required for studying individual unlabelled nanosystems in solution, we use signal enhancement in a fiber-based Fabry-Perot microcavity with high finesse (F~105 ), which is integrated into a microfluidic channel. The presence and dynamics of a nanoobject in the tight focus of the cavity mode (w0 ~1μm) can be detected via the induced frequency shift and decrease of amplitude of the resonant cavity transmission signal, resulting from the excess polarizability of the object. For smaller nanoobjects, the sensitivity can be increased by the active stabilization of the cavity length and the interferometric measurement of the phase shift induced by the nanoparticle (homodyne detection). Shifting the signal to a higher frequency (heterodyne detection) leads to a further increase of the sensitivity.
Besides the determination of the size and the refractive index of the nannoobject, also their position can be tracked by changing the cavity length at high frequency and measuring the resonance frequency and linewidth of several transverse modes of the cavity. Due to the different radial shape of cavity transverse modes, this yields information about the spatial position of the nanoobjects, allowing us to track the position with high temporal resolution. [Kohler et al., Nature Commun. (2021)]
Cavity-enhanced spectroscopy of single-wall carbon nanotubes
Carbon nanotubes (CNTs) offer an intriguing combination of optical, electronic, and mechanical properties. We develop different techniques of cavity-enhanced spectroscopy to characterize individual CNTs. The figure shows exemplary spatially resolved, cavity-enhanced Raman spectroscopy to obtain spatial maps of molecular-fingerprint information (right image). At the same time, cavity-enhanced absorption microscopy allows us to quantitatively determine the extinction cross section (left image). Investigation of the details of Raman modes gives insight into electronic and structural properties. (Hümmer et al., Nature Commun. (2016)). In a different direction, localized excitons in CNTs can be useful as quantum light source. We work on the Purcell enhancement of telecom single photon emission from chemically functionalized CNTs.
Exciton polaritons in two-dimensional semiconductors
Atomically thin crystals of transition metal dichalcogenides (TMDs) host excitons with strong binding energies and sizable light-matter interactions. Coupled to optical cavities, monolayer TMDs routinely reach the regime of strong light-matter coupling, where excitons and photons admix coherently to form quasiparticles known as polaritons up to room temperature. We explore the two-dimensional nature of TMD polaritons with cavity-assisted hyperspectral imaging. We record a spatial map of polariton properties of extended WS2 monolayers coupled to a tunable micro cavity in the strong coupling regime, and correlate it with maps of exciton extinction and fluorescence taken from the same flake with the cavity. We find a high level of homogeneity, and show that polariton splitting variations are correlated with intrinsic exciton properties such as oscillator strength and linewidth. Moreover, we observe a deviation from thermal equilibrium in the resonant polariton population, which we ascribe to non-Markovian polariton-phonon coupling. Our measurements reveal a promisingly consistent polariton landscape, and highlight the importance of phonons for future polaritonic devices. [Gebhardt et al., Sci. Rep. (2019)]
Analogous concepts for hybrid light-matter systems employing spatially indirect excitons with a permanent electric dipole moment in heterobilayer crystals promise realizations of exciton-polariton gases and condensates with inherent dipolar interactions. Here, we implement cavity-control of interlayer excitons in vertical MoSe2-WSe2 heterostructures. Our experiments demonstrate the Purcell effect for heterobilayer emission in cavity-modified photonic environments, and quantify the light-matter coupling strength of interlayer excitons. The results will facilitate further developments of dipolar exciton-polariton gases and condensates in hybrid cavity – van der Waals heterostructure systems. [Förg et al., Nature Commun. (2019)]
Scanning cavity microscopy
Improving the sensitivity of optical microscopy could give access to the optical properties of a large class of interesting nanoscale systems on a single particle level. To boost sensitivity, we are developing a novel scanning microscopy technique that harnesses multiple interactions of probe light with an object. This is realized by placing the sample inside a high-finesse optical microcavity, which provides a signal enhancement on the order of the cavity Finesse (104 – 105). Raster-scanning the sample through the cavity mode enables us to obtain spatial maps of sample absorption, dispersion, and birefringence of individual nanoparticles.
The method should enable e.g. the spectroscopic characterization of individual nanoparticles, dispersive sensing with high spatial resolution, and the detection of non-fluorescent single molecules. [Mader et al., Nature Commun (2015), Hümmer et al., Nature Commun. (2016), Mader et al., ACS Photonics (2022)].