Research topics
One of the central goals is to enhance light-matter interactions to realize efficient light-matter interfaces at the single quantum level, and to enable novel schemes for spectroscopy and sensing. We employ and further develop fiber-based Fabry-Perot microcavities, which combine microscopic mode volumes with exceptionally high quality factors, and at the same time offer open access for a variety of samples.
Spin-photon interface for single rare earth ions
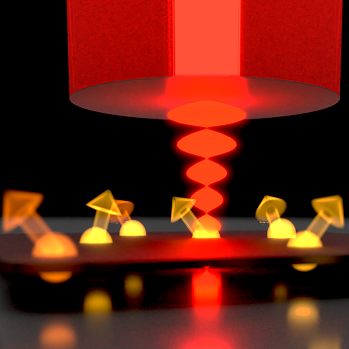

Rare earth ions provide exceptional optical and hyperfine coherence, which makes them promising candidates for quantum information applications, ranging from quantum memories and quantum computing nodes to quantum-nonlinear optics. However, the dipole-forbidden nature of the narrow transitions results in ultra-low emission rates, limiting most experiments to macroscopic ensembles. We want to gain efficient access to individual ions and small ensembles by coupling them to a high finesse optical microcavity. Purcell enhancement can boost the emission rate by several orders of magnitude, thereby making the weak transitions bright and enabling efficient state readout. We use microcavities based on laser-machined optical fibers as mirror substrates, which combine high finesse with small mode cross sections and full tunability.
The fiber-opposing planar mirror incorporates the rare-earth ion doped nanocrystals in the cavity (Fig.1). Addressing of individual ions is achieved in the spectral domain via a stabilized, narrowband laser. This scheme makes use of the large ratio between the broad inhomogeneous crystal linewidth versus a narrow homogeneous single ion linewidth (Fig.2) opening up the possibility to have efficient access to possibly thousands of qubits contained in one cavity mode.
Spin Photon interface for color centers in diamond
Building a long distance quantum network is one of the big challenges in the field of quantum communication, which requires the development of a quantum repeater. A crucial component of this is an efficient, coherent spin photon interface, and coupling single color centers in diamond to a microcavity is a promising approach therefore. In our experiments, we integrate a diamond membrane to an open access fiber-based Fabry-Perot microcavity to attain emission enhancement into a single well-collectable mode as well as spectral filtering. Simulations predict the feasibility of a strong enhancement of the Zero-Phonon-Line (ZPL) emission efficiency, reaching values of up to 80%. We are working on spatially resolved characterizations of different coupled cavity-membrane devices and on the integration of such devices in a cryogenic environment.
Cavity-enhanced sensing platform in a microfluidic cell
The detection and characterization of individual non-fluorescent nanosystems with high temporal resolution could provide new insights into their intrinsic properties and dynamics. To achieve the high sensitivity required for studying individual unlabelled nanosystems in solution, we use signal enhancement in a fiber-based Fabry-Perot microcavity with high finesse (F~105 ), which is integrated into a microfluidic channel. The presence and dynamics of a nanoobject in the tight focus of the cavity mode (w 0 ~1μm) can be detected via the induced frequency shift and decrease of amplitude of the resonant cavity transmission signal, resulting from the excess polarizability of the object. For smaller nanoobjects, the sensitivity can be increased by the active stabilization of the cavity length and the interferometric measurement of the phase shift induced by the nanoparticle (homodyne detection). Shifting the signal to a higher frequency (heterodyne detection) leads to a further increase of the sensitivity.
Besides the determination of the size and the refractive index of the nannoobject, also their position can be tracked by changing the cavity length at high frequency and measuring the resonance frequency and linewidth of several transverse modes of the cavity. Due to the different radial shape of cavity transverse modes, this yields information about the spatial position of the nanoobjects, allowing us to track the position with high temporal resolution. Also other spectroscopic technics can be exploited. E.g. absorption spectroscopy gives information about the absorption cross section of the nanoobject independent to its scattering cross section. If the cavity length is actively stabilized, the electric dipole forces can be used to trap the nanoparticle inside the cavity.
Cavity-enhanced spectroscopy of single-wall carbon nanotubes
Carbon nanotubes (CNTs) offer an intriguing combination of optical, electronic, and mechanical properties. We develop different techniques of cavity-enhanced spectroscopy to characterize individual CNTs. The figure shows exemplary spatially resolved, cavity-enhanced Raman spectroscopy to obtain spatial maps of molecular-fingerprint information (right image). At the same time, cavity-enhanced absorption microscopy allows us to quantitatively determine the extinction cross section (left image). Investigation of the details of Raman modes gives insight into electronic and structural properties. (Hümmer et al., Nature Commun. (2016)). In a different direction, localized excitons in CNTs can be useful as quantum light source. We work on the Purcell enhancement of telecom single photon emission from chemically functionalized CNTs.
Exciton polaritons in two-dimensional semiconductors
Atomically thin crystals of transition metal dichalcogenides (TMDs) host excitons with strong binding energies and sizable light-matter interactions. Coupled to optical cavities, monolayer TMDs routinely reach the regime of strong light-matter coupling, where excitons and photons admix coherently to form quasiparticles known as polaritons up to room temperature. We explore the two-dimensional nature of TMD polaritons with cavity-assisted hyperspectral imaging. Using extended WS2 monolayers, we establish the regime of strong
Scanning cavity microscopy
Improving the sensitivity of optical microscopy could give access to the optical properties of a large class of interesting nanoscale systems on a single particle level. To boost sensitivity, we are developing a novel scanning microscopy technique that harnesses multiple interactions of probe light with an object. This is realized by placing the sample inside a high-finesse optical microcavity, which provides a signal enhancement on the order of the cavity Finesse (104 – 105). Raster-scanning the sample through the cavity mode enables us to obtain spatial maps of sample absorption, dispersion, and birefringence of individual nanoparticles.
The method should enable e.g. the spectroscopic characterization of individual nanoparticles, dispersive sensing with high spatial resolution, and the detection of non-fluorescent single molecules. [Mader et al., Nature Commun (2015)].